Methane is a greenhouse gas (GHG) that contributes to global warming. If we are to manage our global inventory of GHG’s, we must learn to balance the concentration of methane in the atmosphere, along with other GHG’s like carbon dioxide (CO2). Balancing methane concentration requires that we understand both the risks and potential benefits of the role of methane in our ever-evolving net-zero energy system.
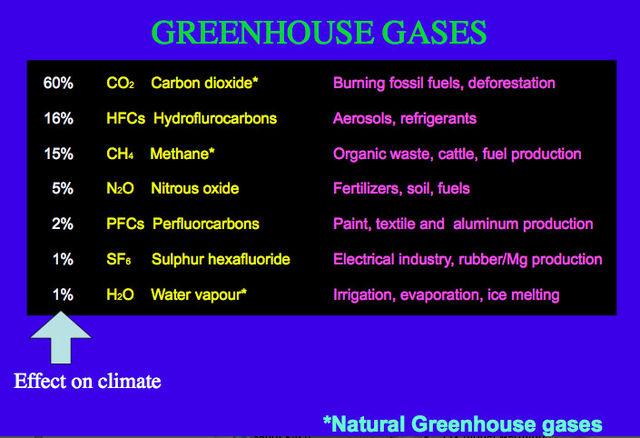
In this article, we will attempt to re-frame the discussion about the future of methane in all its forms and sources. In future articles, we’ll dig into the details of some of the ideas presented here. For our society to prosper and thrive while meeting our environmental goals we believe that methane must be able to play an essential role going forward.
The Traditional Way of Thinking About Methane
Methane is traditionally thought of as an energy source produced by drilling into the ground whose value is primarily gained via combustion, thereby producing atmospheric CO2 as a by-product. (This ignores its use in making fertilizer, plastics, and other chemicals, but this is not the primary focus of this discussion.) Because it is produced from finite, geologic sources, its cost will be ever increasing as supplies become harder to extract.
Methane is most economical and practical when it is transported via underground pipeline and stored for seasonal use in numerous ways, but especially in certain natural underground “reservoirs”. Other methods of shipping methane as a liquid (also known as LNG) or highly compressed gas (CNG) can also be economically attractive, especially where methane’s clean environmental profile is valued (compared to coal, for example, for producing electricity). Finally, methane can be very attractive as a clean, inexpensive transportation fuel (especially for trucks).
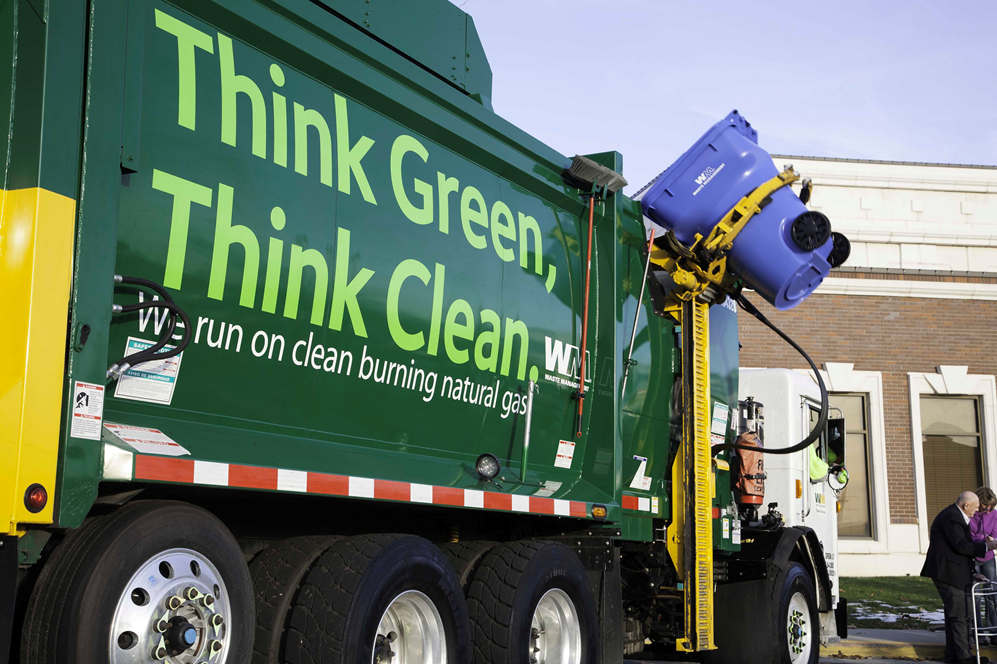
How We Should be Thinking of Methane as we Move to a Net-Zero Energy System
Methane is an industrial chemical/energy system component that serves as a low/zero carbon energy source as well as a clean/renewable energy carrier and storage system. It is a molecule that occurs naturally but can also be produced at industrial scale.
Methane can be produced at an industrial scale by taking carbon dioxide (extracted from the atmosphere or other sources) and combining it with hydrogen produced by electrolysis of water. If the electrolysis process is powered by electricity from a carbon-neutral source, such as solar, wind, or nuclear, the resulting methane is also carbon neutral even if it is combusted without carbon capture at the end use.
Natural sources of methane include geologic deposits (i.e., natural gas); but also, many animals, bogs, and swamps; and coal seams (whether mined or not). Among animals, domesticated cattle are frequently cited as a major source, but, in fact, most herbivores from elephants to humans to termites also release methane. Common human commercial infrastructure, such as landfills and wastewater treatment plants, also release methane. Clearly, we cannot stop all methane releases into the atmosphere, so the issue is how they are managed to meet environmental and economic goals.
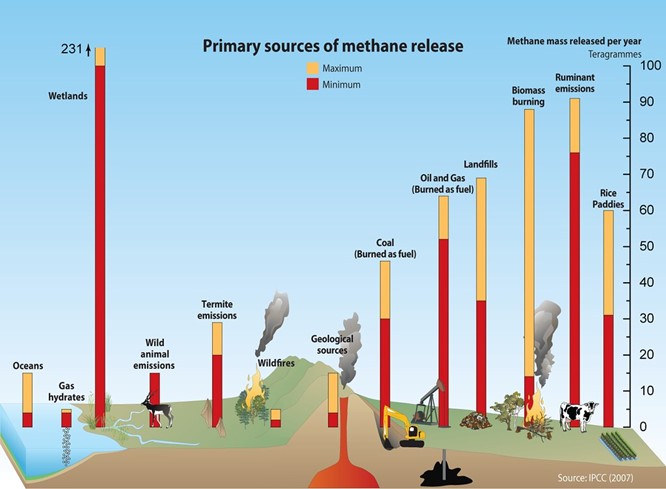
Methane can be a zero carbon, and even “negative” carbon, when envisioned as part of a net-zero energy system depending on its source and the technology through which the methane is converted to useful energy (such as heat, mechanical power, and/or electricity). Just as electricity can be carbon intensive or carbon neutral, based on how it is produced and used, so is the case with methane.
Carbon Negative Methane
Today, carbon negative methane is widely produced around the world from decomposing organic matter such as agricultural wastes (e.g., dairy manure), landfills, and wastewater treatment plants. Because methane from these sources would otherwise naturally escape into the atmosphere, capturing, cleaning, and shipping this methane in the form of renewable natural gas (RNG) is widely considered to be a form of carbon-negative methane.
Zero-Carbon Methane
Zero-carbon methane can be produced on an industrial scale by combining CO2 from the air with hydrogen that is produced via electrolysis driven by renewable or nuclear energy. In this scenario, the CO2 that is produced when the methane is burned is “recycled” as a carrier of the hydrogen. There are various process schemes that can be employed to produce methane in this general manner. Terms used to describe such zero-carbon methane include e-methane, synthetic natural gas (SNG) and methanated hydrogen. In these energy pathways, methane is simply carrying energy as a molecule, whereas electricity carries energy as an electron.
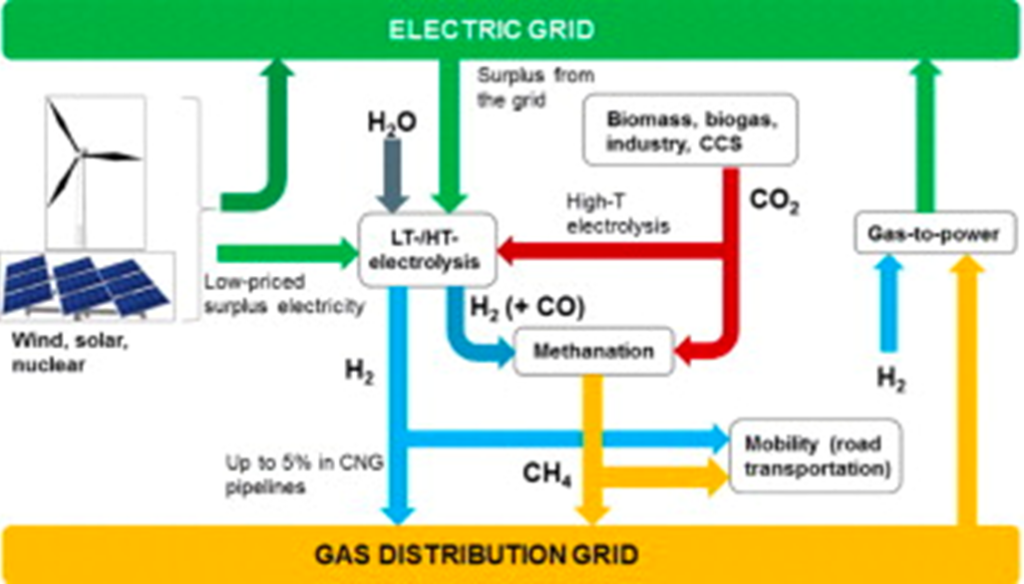
Advantages of Storing Methane vs. Electrical Energy
It is much easier to store methane’s molecular energy, especially longer-term seasonally, than to store electrical (electron) energy. Methane simply needs an impermeable enclosure, such as a naturally occurring, subterranean salt dome or depleted natural gas reservoir that is not subject to significant deterioration over time. The technical reason for this ease of storage is that the methane is stored in original form without needing conversion to other temporary storage media. There are currently over 400 such underground storage facilities in the US. Electricity, in contrast, needs a highly engineered and costly battery, in some cases comprising expensive (frequently toxic and flammable) materials that degrade over time. To be stored in a battery, the electrical energy needs to go through two conversions: from electrical (moving electrons) to chemical then back to electrical. The conversions are expensive and result in energy losses.
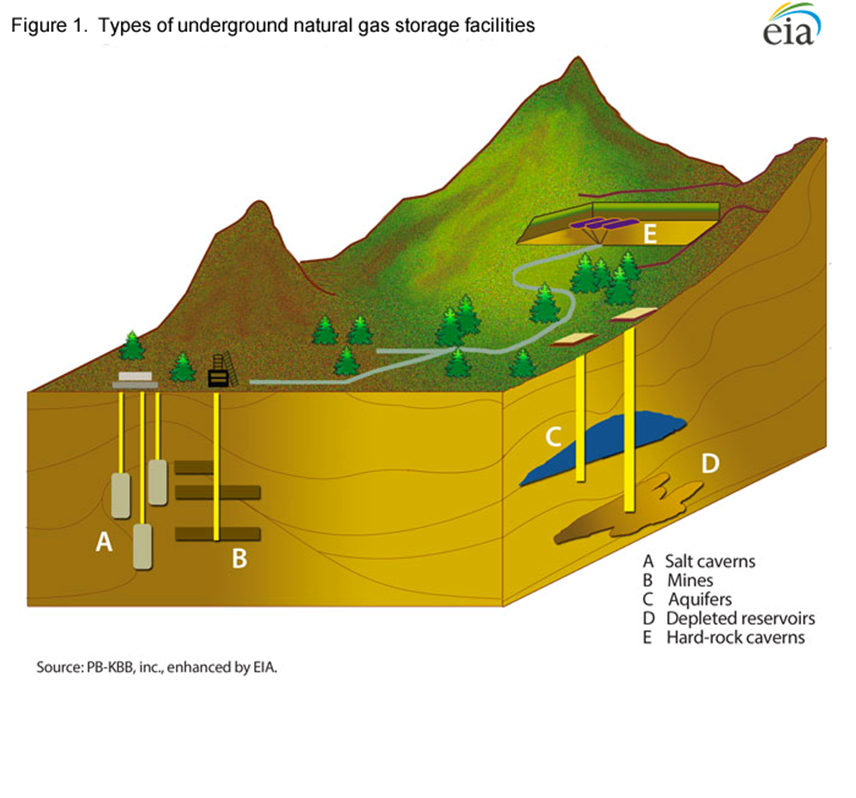
Synthetic Methane Can Transport Hydrogen Energy
There are several reasons why one might want to use synthetic methane to “carry” hydrogen energy:
- Methane is completely compatible with the current gas infrastructure of transmission, distribution, and use. While pure hydrogen can be blended with natural gas in modest proportions (perhaps up to 20%) using the existing gas system, such blending would only partially decarbonize the gas distribution system.
- Synthetic methane could serve industrial processes (e.g., steel, ammonia, cement, chemical industry) that would be hard to decarbonize if electricity were the only option.
- Both hydrogen and methane are less dense than traditional liquid fuels, so, if they are not moved in a pipeline, each would need to be liquified or compressed, for example, for use as a transportation fuel. However, methane can be liquified for transport at a significantly warmer temperature (-160oC) than hydrogen (-253oC). Similarly, compressed to the same high pressure, compressed methane contains more energy than hydrogen. These afford an advantage for methane over hydrogen.
- The technology and infrastructure for long term storage of methane is well established. However, it is not certain that hydrogen could utilize these same technologies and facilities due to its greater diffusivity (the ability to penetrate through, for example, the walls of an underground reservoir). This storage capability opens the door for large scale, seasonal storage of hard-to-forecast renewable energy, such as wind or solar.
In thinking about such “new methane” scenarios, it can be helpful if we simply think of methane as just another industrial chemical. Like most industrial chemicals, it affords benefits to society, but also risks. One could list hundreds, if not thousands, of other chemicals that fit this description: lead, mercury, alcohols, benzines, chlorine, radio-active isotopes, thousands of drugs…. as well as various sources of radiation such as X-rays and UV light. These chemicals are regulated to ensure their social benefits outweigh their risks. We need to start to view methane, either synthetic or natural, in the same way.
For this reason, our vision of methane in the future needs to be:
manage it, don’t ban it.
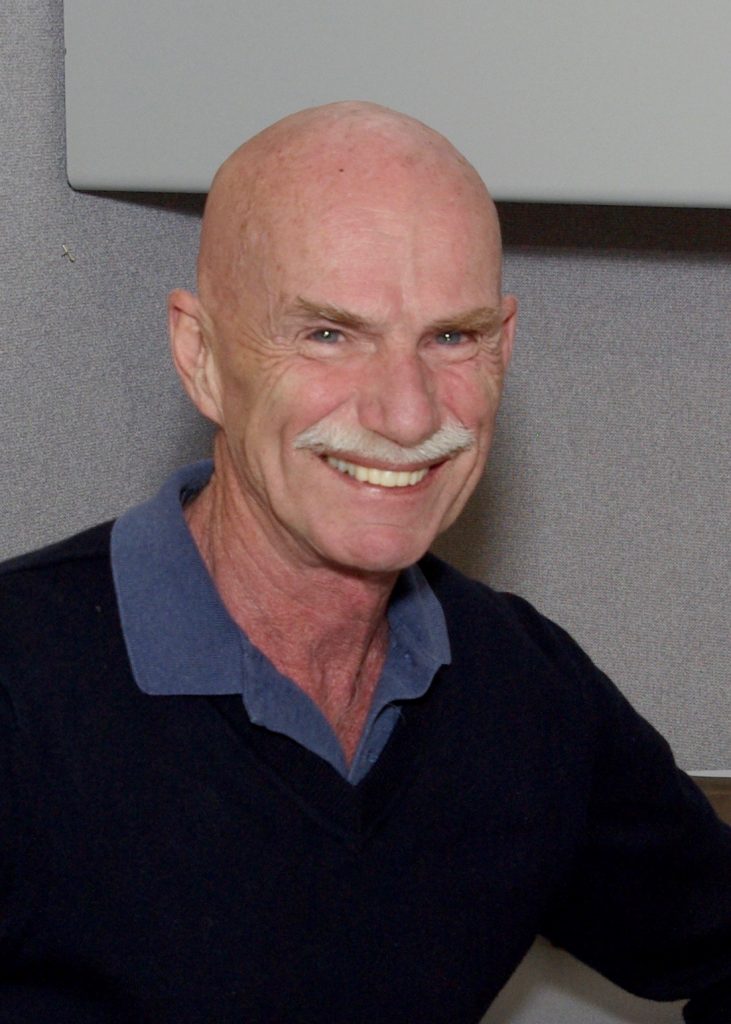
Jeff is the Technical Advisor/Co-founder of Onboard Dynamics. He is an experienced entrepreneur, having founded or co-founded two companies in the energy and software industries before co-founding Onboard Dynamics.